Archaeotechnology
The Key Role of Impurities in Ancient Damascus Steel Blades
J.D. Verhoeven, A.H. Pendray, and W.E. Dauksch
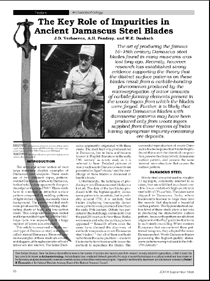 |
CONTENTS |
---|
|
The art of producing the famous 16-18th century Damascus steel blades found in many museums was lost long ago. Recently, however, research has established strong evidence supporting the theory that the distinct surface patterns on these blades result from a carbide-banding phenomenon produced by the microsegregation of minor amounts of carbide-forming elements present in the wootz ingots from which the blades were forged. Further, it is likely that wootz Damascus blades with damascene patterns may have been produced only from wootz ingots supplied from those regions of India having appropriate impurity-containing ore deposits.
Author's Note: All compositions are given in weight percent unless otherwise noted.
The arms and armor section of most large museums display examples of Damascus steel weapons. These steels are of two different types, pattern-welded Damascus and wootz Damascus, both of which were apparently first produced prior to around 500.1,2 These steels have in common an attractive surface pattern composed of swirling patterns of light-etched regions on a nearly black background. The pattern-welded steels were produced by forge welding alternating sheets of high- and low-carbon steels. This composite was then folded and forge-welded together, and the fold/forge cycle was repeated until a large number of layers was obtained.
This article is concerned with the second type of Damascus steel, sometimes called oriental Damascus. The most common examples of these steels are swords and daggers, although examples of body armor are also known. The name Damascus apparently originated with these steels. The steel itself was produced not in Damascus, but in India and became known in English literature in the early 19th century3 as wootz steel, as it is referred to here. Detailed pictures of many such wootz Damascus swords are presented in Figiel's book,4 and the metallurgy of these blades is discussed in Smith's book.5
Unfortunately, the technique of producing wootz Damascus steel blades is a
lost art. The date of the last blades produced with the highest-quality damascene
patterns is uncertain, but is probably around 1750; it is unlikely that blades
displaying low-quality damascene patterns were produced later than the early
19th century. Debate has persisted in the metallurgy community over the past
200 years as to how these blades were made and why the surface pattern appeared.6-8
Research efforts over the years have claimed the discovery of methods to reproduce
wootz Damascus steel blades,9-12 but all of these
methods suffer from the same problemmodern bladesmiths have been unable
to use the methods to reproduce the blades. The successful reproduction of wootz
Damascus blades requires that blades be produced that match the chemical composition,
possess the characteristic damascene surface pattern, and possess the same internal
microstructure that causes the surface pattern.
Wootz steel was produced as roughly 2.3 kg ingots, commonly referred to as cakes, that are solidified in a closed crucible. It was a relatively high-purity iron steel with 1.5% carbon. The cakes were shipped to Damascus, Syria, where bladesmiths learned to forge them into the swords that displayed a beautiful surface pattern. The hypereutectoid carbon level of these steels plays a key role in producing the characteristic surface pattern, because the pattern results from alignment of the Fe3C particles that form in such steels on cooling. When western Europeans first encountered these patterned weapons, they adopted the name Damascus steel. Wootz Damascus blades possessing the highest-quality damascene patterns were produced in the 16th-17th century.4
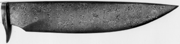 |
a |
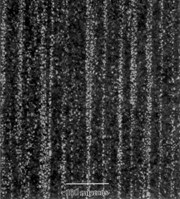 |
b |
Figure 1. (a) A reconstructed wootz Damascus blade showing the Damascene surface pattern containing a combined Mohammed ladder and rose pattern. (b) A longitudinal section of the same blade showing the bands of cementite particles responsible for the surface pattern. |
Both the internal microstructure and the chemical composition of these steels were well established early in this century.11,13 The internal microstructure of a wootz Damascus blade possessing a high-quality damascene surface pattern is a unique metallurgical microstructure.8 It consists of bands of small (generally around 6 mm diameter) particles of Fe3C (cementite) clustered along the band centerline. The bands have a characteristic spacing in the 30-70 mm range and are contained in a steel matrix. The structure of the steel matrix varies depending on how the smith heat-treated the blade, but it is generally found to be pearlite. The bands lie parallel to the forging plane of the blades. By manipulating the angle of the blade surface relative to the plane of the bands, the smith can produce a variety of convoluted patterns of intersection of the bands with the blade surface. With polishing and etching, the Fe3C particles cause the bands to appear white and the steel matrix nearly black; thus, the surface pattern is created.
In recent work, a technique to produce blades that match the best museum-quality wootz Damascus blades in both surface appearance and internal microstructure has been developed. Figure 1 presents a blade recently made by one of the authors, A.H. Pendray, showing the characteristic damascene surface pattern. It has been specially prepared to include the famous Mohammed's ladder pattern that appears on many of the higher-quality museum swords and blades. The circular pattern between the ladders is often termed the rose pattern, and it is also sometimes found on high-quality museum blades.4 A longitudinal section from an adjoining piece of this blade is also shown, which illustrates the aligned bands of clustered cementite particles typical of the better quality museum blades.
A detailed picture description of the production process for this blade has recently been published.14 In addition, the technique has been fully described in the literature,15-17 and it has been shown that blades possessing high-quality damascene patterns can be repeatedly produced utilizing the technique. The technique is, in essence, a simple reproduction of the general method described by the earlier researchers. A small steel ingot of the correct composition (Fe + 1.5C) is produced in a closed crucible and is then forged to a blade shape. However, some key factors are now specified. These include the time/temperature record of the ingot preparation, the temperature of the forging operations, and the type and composition level of impurity elements in the Fe + 1.5C steel. It appears that the most important factor is the type of impurity elements in the steel ingot. Recent work17-18 has shown that bands of clustered Fe3C particles can be produced in the blades by the addition of very small amounts (0.03% or less) of one or more carbide-forming elements, such as V, Mo, Cr, Mn, and Nb. The elements vanadium and molybdenum appear to be the most effective elements in causing the band formation to occur. An obvious question raised by these results is, are these elements also present at low levels in the 16-18th century wootz Damascus blades?
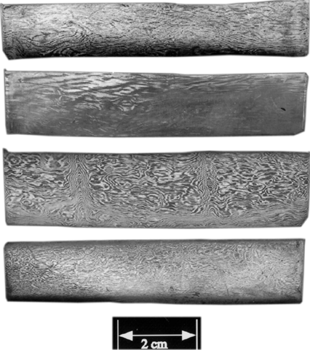 |
Figure 2. Macrophotographs of Zschokke sword blades. |
A major problem in doing scientific experiments on wootz Damascus steel is the inability to obtain samples for study. Such study requires that the blades be cut into sections for microscopic examination, and small quantities must be sacrificed for destructive chemical analysis. A rare example where museum-quality wootz Damascus blades were donated to science for study is reported in the 1924 paper of Zschokke.13 A famous explorer and collector, Henri Moser, amassed a collection of some 2,000 Damascene blades and donated two daggers and four swords to Zschokke for study. The Moser collection is now displayed in the Berne Historical Museum in Switzerland, and the remaining pieces from the four swords of the Zschokke study remain there. Recently, Ernst J. Kläy of the Berne Museum donated a small sample of each sword for further study to be conducted.
This article presents the results of a study of these four samples. Also, four additional wootz Damascus blades, all thought to be a few hundred years old, have been acquired and are included. Hence, all of the blades studied here are more than two centuries old and were presumably made from wootz steel. These blades are referred to as genuine wootz Damascus blades to differentiate them from the reconstructed wootz Damascus blades made by the technique developed by the authors.
Zschokke identified the four swords of his study as swords 7-10, and the same code is used here. The swords had an original width of around 30 mm. The samples provided were approximately 18 mm wide by 88 mm in length and contained the cutting edge. The surface of the samples were refinished by polishing with fine SiC papers and then etching in ferric chloride. The contrast on the sample's surface was enhanced by applying the ferric chloride with repeated rubbing from a cloth. Figure 2 presents macrographs of the four sword samples; sword 9 has the most distinct pattern.
Pieces were cut from one end of each of the samples with a thin diamond saw. A 2 cm length was cut for chemical-analysis studies, and an 8 mm length sample was used for microstructure analysis. The chemical analyses were done using emission spectroscopy on a calibrated machine at Nucor Steel Corporation. Table I presents the chemical analyses, along with the values reported by Zschokke. Agreement between the analyses done by Zschokke in 1924 and the present data is reasonably good.
Table I. A Comparison of the Current Chemical Analyses with Zschokke's Analyses13* |
---|
|
Sword 7 |
Sword 8 |
Sword 9 |
Sword 10 |
Material |
Current |
Zschokke |
Current |
Zschokke |
Current |
Zschokke |
Current |
Zschokke |
C |
1.71 |
1.87 |
0.65 |
0.60 |
1.41 |
1.34 |
1.79 |
1.73 |
Mn |
150 |
50 |
1,600 |
1,590 |
<100 |
190 |
300 |
280 |
P |
1,010 |
1,270 |
1,975 |
2,520 |
980 |
1,080 |
1,330 |
1,720 |
S |
95 |
130 |
215 |
320 |
60 |
80 |
160 |
200 |
Si |
350 |
490 |
1,150 |
1,190 |
500 |
620 |
500 |
620 |
* Analyses are given in parts per million by weight, except for C, which is in weight%. |
Sword 8 is hypoeutectoid and, therefore, cannot be a true wootz Damascus steel, because such steels will not form Fe3C particles on cooling. Metallographic examination confirmed this expectation and revealed that the surface pattern seen on this sword (Figure 2) was due to ferrite bands in a pearlite matrix. Therefore, this sword will not be considered to be a genuine wootz Damascus sword in the following discussion.
Micrographs of surface and transverse sections of the remaining three swords are shown in Figure 3. The micrographs of the surfaces are, in effect, taper sections through the bands seen on the micrographs of the section views, and, as expected, the widths of the bands are expanded in the surface views.
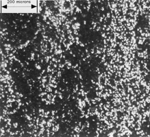 |
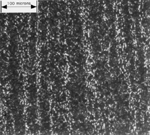 |
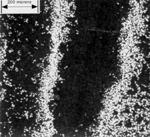 |
a |
b |
c |
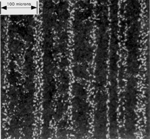 |
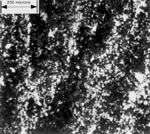 |
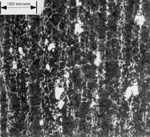 |
d |
e |
f |
Figure 3. Micrographs of Zschokke blades showing (a) the surface of blade 7, (b) a transverse section of blade 7, (c) the surface of blade 9, (d) a longitudinal section of blade 9, (e) the surface of blade 10, and (f) a transverse section of bade 10. |
Table II presents a summary of the microstructural observations of the steels. The three wootz Damascus swords all display band spacing in the 40-50 mm range. Sword 7 contains graphite stringers not shown in the micrographs. Sword 10 contains a mixture of large and small particles in the bands. Sword 9 displays the most distinct bands on the micrographs and also appears to give the most attractive damascene pattern (Figure 2). The bands are the most distinct because this blade contains the least amount of Fe3C particles lying between the carbide bands. Interestingly, however, Zschokke rated the damascening quality of the four swords and reports sword 10 as "the most beautiful and the most precious of the four." Three other museum-quality blades were sectioned,8 and the carbide bands appear similar to sword 9, being more distinct than in swords 7 and 10 and without the large cementite particles of sword 10.
Rockwell C hardness data were taken along the centerline of the transverse sections of all four swords in order to more fully characterize them. A large variation in hardness was found and is presented in Table II. The hardness correlated with the matrix microstructure. The matrix structure of the blades underwent a transition from pearlite at the thin tip to a divorced eutectoid ferrite + cementite at the fat end (thickness = 3-4 mm). These structures are consistent with recent kinetic studies of the eutectoid reaction in hypereutectoid steels.19-20 The studies show that in two-phase (austenite + Fe3C) steels, the divorced eutectoid transformation (DET) dominates at slow cooling rates and the pearlite reaction dominates at higher cooling rates; the DET is favored as the density of the Fe3C particles in the transforming austenite increases. Hence, the matrix microstructures indicate that the blades were air-cooled with pearlite dominating near the faster cooling cutting edge. The dominance of the DET matrix structure in swords 7 and 10 probably results from the higher amount of interband Fe3C present in these swords.
Table II. Microstructural and Hardness Data for the Wootz Zschokke Swords |
Sword |
Microstructure |
Hardness Range |
7 |
Diffuse bands of elongated Fe3C particles in matrix. Significant graphite stringers. Band spacing = 42 µm. Matrix: Pearlite extending 7 mm from the cutting edge; remainder = DET |
Rc = 32, Pearlite matrix
Rc = 8, DET matrix* |
9 |
Very distinct bands of Fe3C particles in matrix. Band spacing = 50 µm. Matrix: Pearlite except for a thin DET region near the fat end |
Rc = 23, Pearlite matrix
Rc = 9, DET matrix* |
10 |
Distinct bands of Fe3C particles in matrix. Band spacing = 46 µm. Pearlite extending 3 mm from the cutting edge; remainder = DET |
Rc = 37, Pearlite matrix
Rc = 5, DET matrix* |
* Divorced eutectoid transformed matrix giving Fe3C particles in ferrite. |
Because of the unique historical value of these blades, a fairly careful study was done to characterize the morphology of the carbide particles comprising the bands causing the damascene patterns. The faces on the 2 cm length samples used to perform the emission spectrograph analyses were mounted and polished and etched in picral. These face surfaces, along with both transverse and longitudinal sections of the swords prepared with similar metallography, were then examined with a high-resolution digital camera. Image-analyzing software was used to determine the average area, maximum diameter, and minimum diameter of the Fe3C particles (Table III). Three regions were examined for each reported measurement. An average of the largest 20 unconnected particles in a sample field of 500-600 particles was determined on each region, and the table presents the average of the three average measurements. The results present a quantitative measure for the shape anisotropy of the particles, which is apparent in Figure 3.
In swords 7 and 10, the particles are dominantly plate-shaped with the thin direction aligned in the forging plane of the sword blades. Consequently, the area of the particles on the sword face is generally larger than on the sections. The standard deviation of the data was consistently in the range of 20-25%, so that differences in the areas on the three surfaces are problematic, whereas, the differences in minimum and maximum diameters are significant. For blades 7 and 10, the maximum/minimum aspect ratio of the particles averages around three on both transverse and longitudinal sections and around two on the sword faces. The ratios are slightly less for blade 9, reflecting the more globular shape of the particles and the observation that the oblong particles do not have their broad face well aligned in the forging plane, as they do on blades 7 and 10.
Table III. A Summary of Fe3C Particle Size Measurements* |
|
|
Section |
Sword |
Dimension |
Face |
Longitudinal |
Transverse |
7 |
Diameter (max./min.) Area |
13/7.4 88 |
16/4.6 69 |
10/3.230 |
9 |
Diameter (max./min.) Area |
11/5.7 59 |
12/5.6 65 |
11/3.9 41 |
10 (small) |
Diameter (max./min.) Area |
13/6.6 76 |
16/4.8 62 |
15.4.9 63 |
10 (large) |
Diameter (max./min.) Area |
54/27 1,300 |
44/14 590 |
46/15 640 |
Kard Blade |
Diameter (max./min.) Area |
8.0/4.0 30 |
|
|
* Diameter is measured in mm; area in mm2. |
The large particles in blade 10 present a significantly larger area on the blade face and might be expected to enhance the damascene pattern. This enhancement was not found on the sample repolished for this study. However, it is difficult to cause the matrix to etch dark on blades 7 and 10 because of the large amount of ferrite produced by the DET structure of the matrix in these blades. With a picral etch, the blade surfaces show a very weak pattern due to this problem, as opposed to a brilliant pattern on blade 9 with its pearlite matrix. With the rubbing ferric chloride etch, the matrix etches darker, but still not as dark as blade 9, as seen in Figure 2. It may be that Zschokke, who had refinished the blades for his 1924 study, employed a superior etching technique that was able to etch the DET matrix of blade 10 darker and thus produce more distinct bands, leading to the conclusion that its damascene pattern was superior to blade 9.
In order to obtain a better statistical sampling of the level of impurity elements in genuine wootz Damascus blades, four additional blades were analyzed. Three of the blades were previously studied, with section views showing well-aligned bands of Fe3C particles similar in morphology to sword 9. Also, the surfaces of all of the blades displayed excellent damascene patterns. The three blades have been identified as Voigt,21 Figiel,8 and Old B.15 All three were reanalyzed for this study on the same emission spectrometer used for the Zschokke swords. The results of the analyses, plus the full analyses of the four Zschokke swords, are presented in Table IV.
Table IV. Chemical Analysis of Seven Wootz Damascus Blades* |
Element |
7 |
9 |
10 |
Old B |
Figiel |
Voigt |
Kard |
C |
1.71 |
1.41 |
1.79 |
1.51 |
1.64 |
1.00 |
1.49 |
Mn |
150 |
<100 |
300 |
100 |
200 |
500 |
100 |
P |
1,010 |
980 |
1,330 |
950 |
1,620 |
260 |
1,440 |
S |
95 |
60 |
160 |
53 |
85 |
115 |
90 |
Si |
350 |
500 |
500 |
470 |
460 |
975 |
500 |
Ni |
600 |
400 |
700 |
<100 |
180 |
<100 |
200 |
Cr |
<100 |
<100 |
<100 |
<100 |
<100 |
<100 |
<100 |
Mo |
<100 |
<100 |
<100 |
<100 |
<100 |
<100 |
<100 |
Cu |
1,750 |
900 |
1,830 |
330 |
780 |
300 |
900 |
Al |
<10 |
<10 |
10 |
12 |
8 |
25 |
30 |
V |
145 |
50 |
270 |
40 |
40 |
<10 |
60 |
Nb |
<100 |
<100 |
<100 |
<100 |
<100 |
<100 |
<100 |
Pb |
<10 |
<10 |
<10 |
<10 |
10 |
10 |
40 |
Sn |
<10 |
10 |
<10 |
<10 |
<10 |
15 |
<10 |
Ti |
9 |
11 |
6 |
13 |
16 |
7 |
19 |
Zr |
<10 |
<10 |
<10 |
<10 |
<10 |
<10 |
<10 |
B |
<1 |
<1 |
<1 |
<1 |
2 |
<1 |
<1 |
Ca |
19 |
17 |
15 |
11 |
2 |
13 |
<1 |
*All analyses are in parts per million by weight, except C, which is in weight percent. |
The kard blade referenced in Tables III and IV is a knife in the style of a Persian kard recently obtained from L. Figiel. It has a walrus-ivory handle (known as shamoni) and chisel work on the blade surface that adjoins the handle, as shown on Figure 4. It was acquired in India by Figiel and is believed to be a genuine wootz Damascus blade produced in the 18th century. This blade was studied to expand the database and illustrate that it is possible to obtain chemical-analysis data using emission-spectrometer analysis without the permanent loss of the damascene pattern. The electric arc utilized in this analysis produces a discolored crater-shaped disk about 1 cm in diameter on the surface in which the surface atoms are vaporized and the pattern destroyed. To successfully arc the blade, it is necessary to clean both sides of the blade surrounding the crater region with emery paper to achieve adequate electrical contact. Figure 4a shows the surface of the kard blade after it had been lightly polished with emery paper and sparked in the emission spectrometer. It was subsequently polished with emery paper to remove the shallow crater region; then, the surface was re-etched with the rubbing/ferric chloride technique.
 |
a |
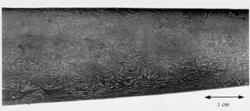 |
b |
Figure 4. (a) The surface of a kard blade showing the emery-paper scratches and the burn mark made by the emission spectrograph analysis. (b) The region near the burn mark after refinishing. |
After polishing, the effects of emission-spectrometer analysis are no longer apparent (Figure 4b). The original damascene pattern has been restored, and, even knowing where the crater had been, it is very difficult to detect its presence by visual examination. The damascene pattern in this blade is well formed, but requires a small magnification to be clearly seen, because of the combination of the small size of the cementite particles and the large amount of interband Fe3C particles in this blade. Digital micrographs of the surface of the kard blade were examined with the same image-analyzing technique used for the Zschokke blades. The results presented at the bottom of Table III illustrate the significantly smaller area of the Fe3C particles in the kard blade versus the Zschokke blades.
The museum-quality wootz Damascus blades with the most attractive surface patterns often display the Mohammed's ladder pattern, similar to that of the blade in Figure 1 and Zschokke sword 9 in Figure 2. Several theories evolved as to how these ladder patterns were produced. Early authors, such as Zschokke,13 upheld a theory attributed to Tschernoff that they resulted from radial dendrites in the steel cakes that were aligned across the blades by piercing the ingots and opening them up during the initial stages of forging. Smith5 argues that this process is unlikely and suggests that they were produced by cutting or grinding shallow grooves across a nearly finished blade and then forging the blade flat, a technique he attributes to Massalski22 and De Luynes.23 Panseri carried out experiments on pattern-welded blades in which he produced transverse grooves in a nearly finished blade by both cutting and forging with a chisel-shaped die.24 He showed that both techniques produce a ladder pattern and argued that the patterns from forged grooves more closely resemble the patterns in wootz Damascus blades than those from cut grooves. This question of how the ladder patterns were produced is also discussed by Figiel,4 who presents several excellent photographs of various ladder-patterned blades.
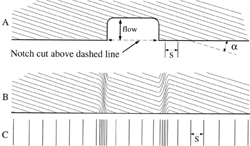 |
Figure 5. (a) A longitudinal section view after a notch cut across blade-(b) Distortion of the carbide bands by forging flow. (c) A schematic of the blade surface showing band spacing after forging flow. |
A qualitative illustration of the mechanism of ladder formation using the notch-cutting technique is presented in Figure 5. For a given band spacing in the blade, the band spacing on the blade surface, S, is controlled by the angle of the band planes with the blade surface, shown as a on Figure 5. When a is increased, the band spacing on the surface decreases. It is the systematic variation of this angle a that results in the oscillation of the spacing of the bands on the blade surface and causes the characteristic wavy damascene pattern. Experiments show that the waviness of the pattern can be dramatically increased by simply using rounded or peening hammer heads to increase variations in a. After cutting a groove across a nearly finished blade, subsequent forging causes the metal at the groove base to fill the groove cavity. The direction of this forging flow is shown by the vertical arrow of Figure 5a. This flow deforms the bands, causing the local a angles at the blade surface to increase at the notch wall locations. The resulting decrease of the surface spacing S at those two locations is shown in Figure 5c. If the grooves are wide, the narrowed band spacing appears along the two walls of the groove and not the bottom, depending on the depth of the groove. The details of the pattern are a complex function of the width and depth of the groove cavity and the shape of the groove cavity.
Experiments have been carried out on the reconstructed wootz Damascus blades in which the ladder and rose pattern were produced by both the groove-cutting and groove-forging techniques. The patterns in the blade of Figure 1 were made with the groove-cutting technique, and detailed photographs of the process have recently been published (Figure 6a).14 These patterns may be compared to similar ladder/rose patterns made by the die-forging technique (Figure 6b). The circular pattern in Figure 6b (called the rose pattern on ancient blades) was made with a hollow cylindrical die, while the pattern in Figure 6a was made by removing metal with a specially shaped solid drill. In the case of the die-forged patterns, the ridges produced by the upsetting action of the die were removed with a belt grinder prior to additional forging.
A comparison of the ladder patterns produced by grinding versus forging reveals nearly identical features (Figure 6). Figiel points out that there is a large variation in the pattern in the bands of the several examples presented in his book.4 Hence, this study is only able to conclude that the ancient smiths produced the ladder patterns by making parallel grooves across the surface of nearly finished blades, either by forging or cutting/grinding.
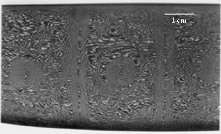 |
a |
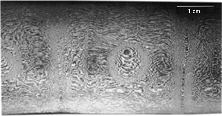 |
b |
Figure 6. The ladder and rose pattern produced by (a) grooves cut into the surface of the nearly finished blade and (b) grooves forged into the surface of the nearly finished blade. |
A major conclusion of the studies on reconstructed wootz Damascus steel17-18 is that the band formation in these steels results from microsegregation of low levels of carbide-forming elements from V, Mo, Cr, Mn, and Nb, with vanadium and molybdenum being most effective. Experiments have shown that vanadium levels as low as 40 parts per million by weight (ppmw) are quite effective in producing the bands of clustered Fe3C particles. The data of Table III show that all of the hypereutectoid steels contain vanadium at or above this level, except for the Voigt blade. However, the Voigt blade contains manganese at the 500 ppmw level, and experiments show18 that banding is induced with manganese levels of only 200 ppmw. Hence, analyses of the seven genuine wootz Damascus steels of Table III are consistent with the theory that low levels of carbide-forming elements, apparently mainly vanadium and to a lesser extent manganese, are essential to the surface-pattern formation of these blades. We believe that it is the microsegregation of these elements during solidification that is causing the Fe3C particles to cluster into bands during the forging, which, in turn, produce the damascene patterns.
It is well established25-28 that the ferrite/pearlite banding of hypoeutectoid steels results from microsegregation of the X element in Fe-C-X alloys, where X is generally manganese, phosphorus, or an alloy addition. For the example X = P, it is established that the microsegregation of phosphorus to the interdendritic regions (IRs) causes ferrite to nucleate preferentially in the IRs. If the cooling rate is slow enough, the ferrite grows as blocky grain boundary allotriomorphs and pushes the carbon ahead of the growth front until pearlite forms between neighboring IRs. Apparently, rolling or forging deformation is quite effective in aligning the IRs of the solidified ingots into planar arrays, because the ferrite appears as planar bands parallel to the deformation plane separated by bands of pearlite. The ferrite/pearlite bands of sword 8 were probably produced by this type of banding caused, most likely, by the microsegregation of phosphorus.
A strong body of evidence has been obtained16-18 that supports the theory that the layered structures in the normal hypereutectoid Damascus steels are produced by a mechanism similar to the mechanism causing ferrite/pearlite banding in hypoeutectoid steels with one important difference in ferrite/pearlite banding, the bands form on a single thermal cycle. For example, the ferrite/pearlite bands can be destroyed by complete austenitization at low temperatures (just above the A3 temperature) followed by rapid cooling and are then reformed in a single heat up to austenite, followed by an adequately slow cool.26 (Low-temperature austenitization is required to avoid homogenization of the microsegregated X element.) The carbide bands of the wootz Damascus steel are destroyed by a complete austenitization at low temperatures (just above the Acm temperature) followed by cooling at all rates, slow or fast. However, if the steel is then repeatedly cycled to maximum temperatures of around 50-100°C below Acm, the carbide bands will begin to develop after a few cycles and become clear after 6-8 cycles.
The formation mechanism of the carbides clustered selectively along the IRs during the cyclic heating of the forging process is not resolved. It seems likely, however, that it involves a selective coarsening process, whereby cementite particles lying on the IRs slowly become larger than their neighbors lying on dendrite regions and crowd them out. A model for such a selective coarsening process has been presented.17 During the heat-up stage of each thermal cycle, the smaller cementite particles will dissolve, and only the larger particles will remain at the forging temperature, which lies just below the Acm temperature. The model requires the segregated impurity atoms lying in the IRs to selectively reduce the mobility of the cementite/austenite interfaces in those regions. Larger particles would then occur in the IRs at the forging temperature. They probably maintain their dominance on cool down because one would not expect the small particles that had dissolved to renucleate on cool down in the presence of the nearby cementite particles. These near-by particles would provide sites for cementite growth prior to adequate local supercooling sufficient to nucleate new particles.
In the course of our research on producing reconstructed wootz Damascus steel blades, it has become evident that it is much easier to forge blades from the small ingots that display little to no damascene pattern than to produce blades with the damascene pattern. The Fe3C particles are still present in these blades, but they are randomly arrayed rather than arrayed as bands. Such blades are widespread in collections and are often referred to as granular blades.4 To produce the banded structure, the right combination of time/temperature firing during ingot making, the right chemical composition (minor element additions), and the proper thermomechanical sequencing during the forging process are required. It is relatively easy to make an ingot that will not pattern on forging.
Based on this experience, it seems likely that the fraction of Indian crucible steel that was successfully forged into the damascened blades was probably quite small; the majority of surviving wootz Damascus blades probably display low-quality surface patterns. Craddock29 has come to this same conclusion based on an analysis of the literature on damascene-patterned steels. The results on the four Moser blades studied by Zschokke support this same conclusion. These blades were supposedly representative of good-quality damascened blades from the east, and yet of the four, only sword 9 displays the high-quality Fe3C bands characteristic of the best museum-quality wootz Damascus blades.
The discovery that vanadium is extremely effective in producing Fe3C banding in high-carbon steels17 was aided by the accidental use of Sorel metal as a raw material for making the small ingots. Sorel metal is a high-purity Fe-C alloy, containing 3.9-4.7% C, marketed by Rio Tinto Iron and Titanium America, Chicago. The alloy is produced from a large ilmenite ore deposit at Lac Tio on the north shore of the St. Lawrence River. Analyses of several batches of the Sorel metal has found that it consistently contains a few hundred ppmw of vanadium impurity. Apparently, the impurity is contained in the ilmenite ore. This suggests the possibility that the low levels of vanadium found in the genuine wootz blades of Table III may have resulted from ore deposits in India where the wootz steels were produced.
One of the big mysteries of wootz Damascus steel has been why the art of making these blades was lost. The vanadium levels provide the basis for a theory. Based on our studies, it is clear that to produce the damascene patterns of a museum-quality wootz Damascus blade the smith would have to fulfill at least three requirements. First, the wootz ingot would have to have come from an ore deposit that provided significant levels of certain trace elements, notably, Cr, Mo, Nb, Mn, or V. This idea is consistent with the theory of some authors30 who believe the blades with good patterns were only produced from wootz ingots made in southern India, apparently around Hyderabad. Second, the data of Table IV confirm previous knowledge that wootz Damascus blades with good patterns are characterized by a high phosphorus level. This means that the ingots of these blades would be severely hot short, which explains why Breant's9 19th century smiths in Paris could not forge wootz ingots. Therefore, as previously shown,15 successful forging would require the development of heat-treating techniques that decarburized the surface in order to produce a ductile surface rim adequate to contain the hot-short interior regions. Third, a smith who developed a heat-treatment technique that allowed the hot-short ingots to be forged might still not have learned how to produce the surface patterns, because they do not appear until the surface decarb region is ground off the blades; this grinding process is not a simple matter.
The smiths that produced the high-quality blades would most likely have kept the process for making these blades a closely guarded secret to be passed on only to their apprentices. The smiths would be able to teach the apprentices the second and third points listed, but point one is something they would not have known. There is no difference in physical appearance between an ingot with the proper minor elements present and one without. Suppose that during several generations all of the ingots from India were coming from an ore body with the proper amount of minor elements present, and blades with good patterns were being produced. Then, after a few centuries, the ore source may have been exhausted or become inaccessible to the smithing community; therefore, the technique no longer worked. With time, the smiths who knew about the technique died out without passing it on to their apprentices (since it no longer worked), so even if a similar source was later found, the knowledge was no longer around to exploit it. The possible validity of this theory could be examined if data were available on the level of carbide-forming elements in the various ore deposits in India used to produce wootz steel.
The authors acknowledge helpful discussions with the late David Peterson and with Rohit Trivedi. Mark Schmidt performed the chemical analyses at the Darlington plant of Nucor Steel Corporation. Hal Sailsbury at Ames Laboratory carried out most of the metallography. The research was funded cooperatively by a grant from Nucor Steel Corporation and the U.S. Department of Energy, Office of Basic Energy Research, through the Ames Laboratory, Iowa State University, contract W-7405-ENG-82.
1. M. Sache, Damascus Steel, Myth, History, Technology Applications (Düsseldorf, Germany: Stahleisen, 1994).
2. B. Bronson, "The Making and Selling of Wootz," Archeomaterials, 1 (1986), pp. 13-51.
3. W. Rostoker and B. Bronson, "Pre-Industrial Iron, Its Technology and Ethnology," Archeomaterial Monograph No. 1 (Philadelphia, PA: Archaeomaterials, 1990), p. 127.
4. L.S. Figiel, On Damascus Steel (Atlantas, FL: Atlantas Arts Press, 1991).
5. C.S. Smith, A History of Metallography, Chapters 3 and 4 (Cambridge, MA: MIT Press, 1988).
6. C.S. Smith, "Damascus Steel," Science, 216 (1983), pp. 242-244.
7. J. Wadsworth and O.D. Sherby, "Damascus Steel-Making," Science, 216 (1983), pp. 328-330.
8. J.D. Verhoeven and D.T. Peterson, "What is Damascus Steel?" Mat. Char., 29 (1992), pp. 355-341.
9. M. Breant, "Description of a Process for Making Damasked Steel," Annals of Philosophy, 8 (1824), pp. 267-271.
10. P. Anossoff and O. Bulatakh, Gornyj Journal (2) (1841), pp. 157-318.
11. N.T. Belaiew, "Uber Damast," Metallurgie, 8 (1911), pp. 449-456; "Damast, seine Struktur und Eigenschaften," Metallurgie, 8 (1911), pp. 699-704; "Damascene Steel," J. Iron and Steel Inst., 97 (1918), pp. 417-439.
12. J. Wadsworth and O.D. Sherby, "On the Bulat-Damascus Steel Revisited," Prog. Mat. Sci., 25 (1980), pp. 35-68.
13. B. Zschokke, "Du Damasse et des Lames de Damas," Rev. Met., 21 (1924), pp. 635-669.
14. J.D. Verhoeven and A.H. Pendray, "The Mystery of the Damascus Sword," Muse, 2 (2) (April 1998), pp. 35-43.
15. J.D. Verhoeven and A.H. Pendray, "Experiments to Reproduce the Pattern of Damascus Steel Blades," Mat. Char., 29 (1992), pp. 195-212.
16. J.D. Verhoeven, A.H. Pendray, and P.M. Berge, "Studies of Damascus Steel Blades: Part IIDestruction and Reformation of the Pattern," Mat. Char., 30 (1993), pp. 187-200.
17. J.D. Verhoeven, A.H. Pendray, and E.D. Gibson, "Wootz Damascus Steel Blades," Mat. Char., 37 (1996), pp. 9-22.
18. J.D. Verhoeven et al., "Microsegregation and Banding in Hypereutectoid Steel: Damascus Steel," ISS Trans., 25 (in press).
19. E.M. Taleff et al., "Pearlite in Ultrahigh Carbon Steels: Heat Treatments and Mechanical Properties," Met. Mat. Trans. A, 27A (1996), pp. 111-118.
20. J.D. Verhoeven and E.D. Gibson, "The Divorced Eutectoid Transformation (DET) in Steel," Met. Mat. Trans. A, 29A (1998), pp. 1181-1189.
21. D.T. Peterson, H.H. Baker, and J.D. Verhoeven, "Damascus Steel, Characterization of One Damascus Steel Sword," Mat. Char., 24 (1990), pp. 355-374.
22. Massalski, "Preparation de l'acier Damasse en Perse," Ann. Du Journal des Mines de Russie (1841), pp. 297-308.
23. H.T.P. J. duc de Luynes, Memoire sur la Fabrication de l'acier Foundu et Damassee (Paris: 1844).
24. C. Panseri, "Damascus Steel in Legend and Reality," Gladius, IV (1965), pp. 5-66.
25. R.A. Grange, "Effect of Microstructural Banding in Steel," Met. Mat. Trans. A, 2 (1971), pp. 417-426.
26. L.E. Samuals, Optical Microscopy of Carbon Steels (Metals Park, OH: ASM, 1980), pp. 154-161.
27. S.W. Thompson and P.R. Howell, "Factors Influencing Ferrite/Pearlite Banding and Origin of Large Pearlite Nodules in a Hypoeutectoid Plate Steel," Mat. Sci. Tech., 8 (1992), pp. 777-784.
28. R. Grossterlinden et al., "Formation of Pearlite Banded Structures in Ferrite-Pearlite Steels," Steel Research, 63 (1992), pp. 331-336.
29. P.T. Craddock, "Cast Iron, Fined Iron, Crucible Steel: Liquid Iron in the Ancient World," Prehistory of Mining and Extractive Metallurgy, ed. P.T. Craddock and J. Lang (London: British Museum, in press).
30. H. Maryon, "Pattern-Welding and Damascening of Sword-Blades-Part 2," J. of Intern. Inst. for Conservation of Hist. and Art Works, 5 (1960), pp. 52-60.
ABOUT THE AUTHORS
J.D. Verhoeven is currently a professor in the Materials
Science and Engineering Department at Iowa
State University. A.H. Pendray is currently president
of the Knifemakers Guild. W.E.
Dauksch is retired as vice president and general manager of Nucor
Steel Corporation.
For more information, contact J.D. Verhoeven, Iowa State University, Materials Science and Engineering Department, 104 Wilhelm Hall, Ames, Iowa 50011; (515) 294-9471; fax (515) 294-4291; jver@iastate.edu.
Copyright held by The Minerals, Metals & Materials Society, 1998
Direct questions about this or any other JOM page to jom@tms.org.